Biomass Overview
It's a tool in the toolkit, but probably not one we want to rely on too heavily
I’d mentioned that I’m trying out a few different illustrators. It’s been great having more than just stock photography to work with. Images this time are by Aarati Asundi, founder of Sykom.
Biomass is frequently touted as an important source of renewable energy. In some published scenarios for net zero emissions, it accounts for 25% or more of total world energy supply – roughly comparable to coal’s share today1. And yet, despite this major projected role, biomass receives comparatively little discussion. In this post, I’m going to present a general overview of the potential of biomass as a clean energy source, explain why it is not necessarily as clean as the prefix “bio” might suggest, and why models probably exaggerate its role in the energy transition.
A side note: subscribers may notice that it has been over a month since my last post. I’ve recently started another blog, on the topic of AI, and in particular the potential risks associated with advanced AIs. The goal of the AI blog is the same as for Climateer: to dive into a complex subject and attempt to explain it in a jargon-free fashion. In the first post, What GPT-4 Does Is Less Like “Figuring Out” and More Like “Already Knowing”, I review some of the key strengths and weaknesses of ChatGPT. Posts here on Climateer will be sporadic for the foreseeable future, though I have at least one more in the pipeline right now.
What Is Biomass?
In the context of energy production, “biomass” simply means any biological material that we can use to produce energy, usually by burning it. It’s a broad category, encompassing a range of materials, processing methods, and end uses, much as the term “fossil fuels” covers a broad range of materials and applications. The following are all examples of biomass energy:
Turning trees into wood pellets to burn in an industrial power plant
Burning municipal waste in an incinerator to generate electricity
Processing cow manure in a “digester” (ugh) to produce methane (natural gas)
Converting farm-grown corn into ethanol to be mixed into the automotive gasoline supply
Cooking s’mores over a campfire
Because the term “biomass” covers so much ground, it’s important not to over-generalize. Each source and application of biomass has different pros, cons, costs, and environmental impacts.
Sources of Biomass
Sources of biomass break down into two categories. Dedicated biomass comes from plants which are grown specifically for this purpose. Trees, non-food crops such as bamboo or switchgrass, and food crops such as corn or soybeans can all be used.
(Readers of this blog may recall that I’ve touched on the subject once before, criticizing the idea of using dedicated biomass to produce electricity, primarily because it requires an exorbitant amount of land.)
Waste biomass encompasses all other sources of biological material used to produce energy. Some significant categories:
Forestry residues (parts of the tree that are left in the forest after logging, such as branches and leaves, as well as entire trees that are too small, diseased, or otherwise unusable for lumber).
Wood processing residues (material that makes it as far as a sawmill or other processing facility, but doesn’t find its way into the final product; for instance, bark, chips, and sawdust). Sometimes referred to as “secondary feedstocks”, as opposed to forestry residues which are “primary feedstocks”.
Agricultural residues (any part of a crop plant that is left on the field after harvesting, such as stalks and leaves).
Animal manure.
Municipal solid waste (garbage, yard trimmings, some industrial waste, scrap wood, etc.).
These materials of course vary widely in availability, geographic distribution, processing requirements, and cost. Even within a particular category, there can be significant variation in size, water content, and other variables.
Applications for Biomass
Proposed uses for biomass primarily break down into four categories:
Burn it to produce electricity (competing with solar, wind, nuclear, and other sources of electricity).
Burn it to produce heat, often for industrial purposes (competing with heat pumps, hydrogen, and other sources of heat).
Convert it into some other fuel, to power ships, airplanes, long-haul trucks, or other vehicles (competing with electric vehicles, and other unconventional fuel sources and types, such as air-to-fuel, hydrogen, or ammonia).
Convert it into other chemicals, for instance to replace petroleum in the manufacture of plastic.
The proposed arguments in favor of biomass for a given use case generally boil down to an argument that solar power, wind power, and other comparatively straightforward sources of clean electricity aren’t applicable because:
They are intermittent (unavailable when the sun isn’t shining and the wind isn’t blowing)
The application can’t easily be made to run on electricity (e.g. high-temperature industrial heat for steel and cement manufacturing; regions with no reliable electricity supply)
Batteries can’t provide sufficient range (e.g. long-haul aviation)
Biomass is not the only solution to these challenges, but it is one of the contenders.
Separate from energy production, biomass can be used as a source of carbon for sequestration (negative emissions); for instance, Charm Industrial is converting corn stalks into “a stable, carbon-rich liquid” and storing it underground.
And of course some forms of biomass contemplated for energy supply can also be used as food, especially animal feed.
How Much Waste Biomass Is Available?
Dedicated biomass has the unfortunate property of requiring significant amounts of land (the theme of my previous biomass post). Waste biomass doesn’t impose additional land requirements, but there is only so much of it available. How much?
It’s hard to find a clear answer. I imagine this is due to the fact that there are so many different types of biomass, each with local variations. Most of the sources we found report on just one part of the picture, such as certain categories of waste biomass or a certain region, and the assumptions are not always clear. Furthermore, the question is not really how much waste biomass exists, it’s how much we might use in practice, which depends on the location, cost and impact of extraction, and how aggressively you believe the source can safely be harvested (I’ll say more about this later).
For what it’s worth, here are some data points we found. All figures are for 2050, unless otherwise mentioned, and are in units of exajoules (EJ) per year.
A recent report from the IEA projects 63 EJ of energy supply from “organic waste streams” and “forest and wood residues”, and states that this is “well below estimates of global sustainable bioenergy potential”.
A different IEA report projects 51 EJ of bioenergy supply from “wastes and residues” in 2030; this roughly agrees with the previous source (which projected 46 EJ in 2030).
A 2011 paper in Biomass and Bioenergy projects 28 EJ from “residues on cropland”, in a “business-as-usual” scenario, whatever that meant in 2011. This seems broadly consistent with the previous figures, since cropland is just one source of waste biomass.
A 2007 paper in Progress in Energy and Combustion Science projects 76-96 EJ from crop and wood residues and wastes (doesn’t appear to consider manure or municipal solid waste).
A 2018 paper in International Journal of Greenhouse Gas Control states that in 2100, incineration of municipal solid waste could generate 32 EJ.
For perspective, the first data point of 63 EJ per year is equivalent to roughly 10% of global energy usage in 20202. So at least in principle, waste biomass could be a significant source of energy.
Price vs. Supply
As for any commodity, the supply of waste biomass will change depending on the price it fetches. The cost of collecting waste biomass varies wildly according to circumstance; expensive sources won’t be tapped unless the price is high.
The research here was difficult to interpret, but just to convey the general idea, here is a figure from a 2015 paper in GCB Bioenergy. This graph projects availability of agricultural and forest biomass residues in 2050 (in EJ again), as a function of price:
As expected, price and supply increase together. A limit emerges at around 45 EJ. At that point, the article explains, “costs increase rapidly due to increases in transport and harvest costs as the marginal supply is increasingly spread out over larger areas”. The article also notes that transportation is the biggest cost component of forest biomass.
This graph chops off at a price of $10 per gigajoule. In terms of electricity, assuming 40% conversion efficiency, that would translate to $90 per megawatt-hour. For comparison, one source puts the fuel cost for a natural gas plant in the US at around $25 to $30 per megawatt-hour. In both cases, that’s just the price for the raw fuel. Building and operating the biomass power plant, and processing the fuel, would further increase the wholesale cost of the resulting electricity.
Carbon Impact of Biomass Fuels
I’d always assumed that biomass is carbon neutral, basically by definition. The carbon atoms came from the atmosphere, because the carbon in a plant originates in the atmosphere; photosynthesis allows plants to break down CO₂ and keep the carbon atom. So when we burn that biomass, we’re just putting the CO₂ back where it started. (There is also animal-based biomass, such as manure, but this ultimately originates in a plant.)
It turns out that there are a few flaws in this idea, as discussed in an article in Scientific American. First of all, by collecting biomass, we might be interfering with a carbon sink. For instance, suppose we collect and burn some forest waste. Without human interference, some of that material might have wound up sequestered in the soil. By collecting it, we’re negating the carbon sink, which is equivalent to introducing new emissions.
Secondly, even if a particular piece of wood was destined to eventually burn or rot, that might have been years down the road. By burning it today, we put that CO₂ into the atmosphere sooner, giving it more time to contribute to the greenhouse effect. Those short-term effects are important, affecting the peak temperature that we’ll reach on the way to net zero emissions, which in turn determines how much harm will result. The impact is especially bad when we log a forest for fuel, as opposed to collecting waste from trees that were being cut down anyway; it may take the better part of a century for the forest to regrow. From the Scientific American article:
“We call it ‘slow in,’ as in it takes a long time for the carbon to accumulate in the forest, and ‘fast out’—you’re burning it so it goes into the atmosphere rapidly,” said Beverly Law, an expert in forest science and management from Oregon State University.
A Natural Resources Defense Council report notes that logging old-growth forest for fuel is especially harmful, as it will be a long time (if ever) before the land returns to a state containing as much carbon as before it was logged. At this point, every remaining acre of old-growth forest on the planet should be protected from any sort of use that disturbs the trees.
The carbon impact can be reduced using BECCS, or BioEnergy with Carbon Capture and Storage. In this process, biomass is burned for energy, and the carbon dioxide exhaust is captured from the smokestack and stored somehow. BECCS is often touted as a renewable energy source with negative emissions, because the carbon starts out in the atmosphere (as discussed earlier) and ends up underground. In practice, it’s not always that simple. As we just saw, “the carbon starts out in the atmosphere” can be an overly simplistic analysis, depending on the source of the biomass. Smokestack CO₂ capture is not 100% effective. Finally, gathering, processing, and transporting the biomass all consume energy and have the potential to generate emissions. So BECCS can provide energy with reduced or even negative CO₂ emissions, but it’s a complex process, involving a lot of overhead and potential side effects.
Other Impacts of Biomass fuels
Carbon emissions aside, biomass usage can have a variety of impacts, depending on the source and how the biomass is used.
Assuming the biomass is eventually burned – whether directly, or after conversion to some sort of fuel – the combustion will likely emit pollutants: not just carbon dioxide, but potentially other greenhouse gases (such as nitrogen oxides and carbon monoxide), as well as particulate emissions.
When we collect forest or agricultural waste material, we may be robbing the soil of important nutrients such as phosphorus and potassium, reducing soil health and productivity, and/or requiring more use of fertilizer. The material that’s removed may also have played a role in protecting soil from erosion. We were unable to come to any clear understanding of how much biomass can safely be harvested, and recommendations seem to be all over the map. For instance, this chart from a paper in the journal “Energy, Sustainability and Society” lists various jurisdictions as requiring anywhere from 10% to 100% of “coarse woody debris” to be left behind when harvesting biomass from forests. Some of this is due to differences in local conditions, but I suspect much of the variation also stems from from differing opinions or out-of-date regulations. Titus et al. write, “most research is site-specific or relatively short-term and cannot necessarily predict how harvesting affects productivity or biodiversity on operational scales, or elsewhere or over the long-term.”
That said, harvesting waste biomass can also have positive effects. One publication notes that there can be positive impacts, such as enhanced seedling growth, or avoiding excessive nitrate accumulation in nearby bodies of water (from “nitrogen-rich logging residues”). Overall, the impacts of biomass harvesting appear to vary wildly according to the location, circumstances, and harvesting practices. From Energy, Sustainability, and Society:
Furthermore, forest biomass can be co-managed with conventional timber to create bioenergy feedstock from material that might otherwise be burned on-site or left to decompose, or removed to reduce fuel loading in fire-prone areas or facilitate replanting of harvested sites. Where there are markets, forest harvest residues can increase the economic value of products from managed forests, and may also stabilize carbon (C) stocks in fire-prone forests and thus prevent conversion of some forest land to other land uses because of the value of these C stocks.
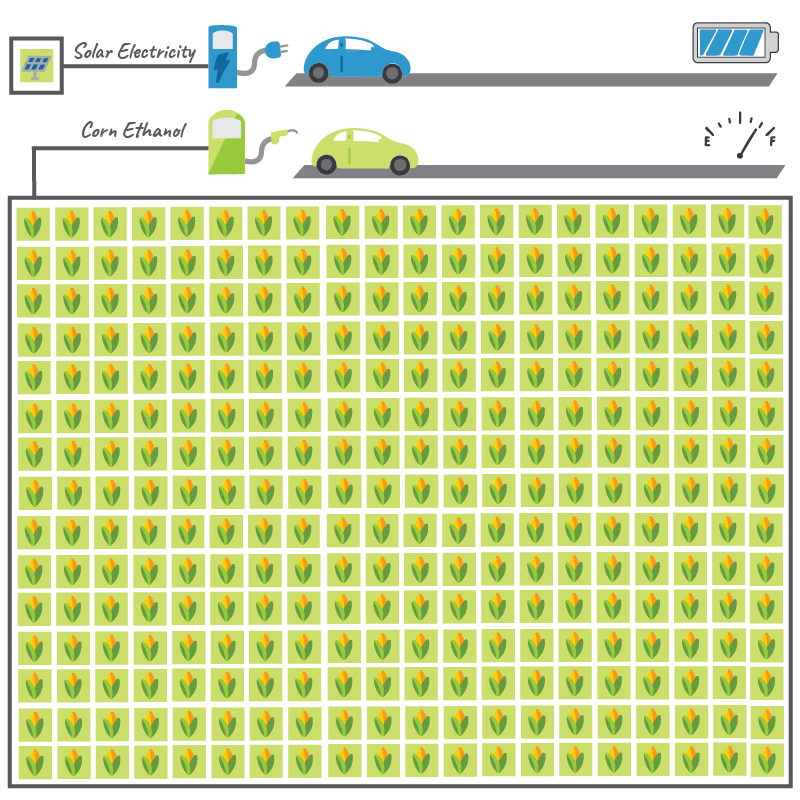
When it comes to dedicated biomass, the overwhelming problem is land usage; especially when arable land is used for biomass crops such as corn or soybeans. As a 2022 article in Canary Media explains, using arable land to grow biomass crops almost certainly results in land being cleared somewhere else to grow more food for human consumption, with the result that biofuels are sometimes even worse for the environment than fossil fuels. If that wasn’t enough, dedicated biomass can also increase use of water (for irrigation) and fertilized. The article also notes that, unfortunately, this type of biofuel consumption often has strong political support from the farming sector.
Problems In Practice
Some sources of renewable energy are comparatively tidy. For instance, rooftop solar panels, once installed, tend to just sit there producing power for years; there is not a lot of complication to the story. It’s true that some mess is involved in procuring the raw materials and producing the panels, but the overall impact over the lifetime of the installation is relatively small.
Biomass energy, on the other hand, is inherently complex and messy. Enormous amounts of material need to be collected, which often involves a certain amount of in-the-field processing (e.g. selecting which forest materials to harvest for lumber, which to collect as waste biomass, and which to leave behind). It then must be transported, often from remote locations, to a facility for further processing, often involving many steps – potentially including sorting and grading, grinding or other preparation, conversion to fuel form, combustion, CO₂ capture and storage, possibly with additional transportation steps along the way.
Biomass is often low-quality fuel, containing substantial amounts of water, soil, or other foreign materials, thus reducing its energy content, increasing transportation and processing costs, and leaving behind wastes that must be disposed of.
This comment from a recent Volts podcast episode on biofuels illustrates the challenges nicely:
We have enough woody biomass to produce all the fuels we need at a cost no one will accept (the tyranny of trucking range is real). So it gets stacked and burned, hauled to the dump and buried, or re-arranged in the forest and the inevitable future fire burns with high intensity despite the work meant to mitigate that intensity. You can't get the feed-stock to the factory, nor can you bring the factory to the feed-stock. It is a frustrating situation, and the inability to get sensible biofuels operating in easy to get to locations like farmlands make the wilderness and forestry story just so much more unlikely.
The upshot of all this is that biomass energy, especially BECCS, looks better on paper than in practice. Depending on the assumptions used, it may be anticipated to be reasonably priced, reliable, and carbon-neutral or -negative. As a result, when a computer model is used to compute an optimal path to net zero emissions, the model will often call for a heavy reliance on BECCS. However, these analyses often suffer from a number of flawed assumptions:
Neglecting that the supply of cheap, safe-to-harvest biomass is limited.
Failure to account for negative secondary effects of biomass use (particulate emissions from combustion, ecological impacts, displacing other uses of land which could ultimately lead to deforestation elsewhere, etc.).
Assuming that BECCS costs will fall at the same rate as wind, solar, and battery power. As we saw last time in Which Technologies Have Learning Curves?, BECCS is the type of technology which typically sees slow price reductions3, while wind, solar, and batteries progress rapidly.
Failing to take into account innovations that reduce the need for chemical fuels, such as alternative steel and cement manufacturing processes; or the potential to use cheap electricity to produce hydrogen or other fuels.
Biomass solutions also provide ample opportunities for greenwashing or outright fraud. For instance, there are documented cases of wood pellets produced from whole oak trees being passed off as “waste”.
Conclusions
The best way to speed the path to zero emissions is to keep as many options open as possible. Different use cases require different solutions, and adding biomass to the menu gives us more options.
That said, I get the strong sense that model scenarios tend to strongly over-favor biomass energy and BECCS. Models are based on quantitative analysis, and BECCS looks better on paper than it is likely to work out in practice, especially if the models ignore secondary effects such as particulate emissions.
I am especially suspicious of dedicated biomass. It takes enormous quantities of material to produce energy, so growing the necessary amount of crops (or trees) requires large amounts of land (often contributing to deforestation), and harvesting, transporting, and processing the material requires large amounts of energy. There may also be significant need for water and fertilizer. When combined with the secondary impacts of biomass use in general – particulate pollution and so forth – it seems very difficult to square the account for dedicated biomass. I’ll finish with a few more data points:
Per a recent episode of the always-excellent Volts podcast, 300 acres of corn can generate enough ethanol to drive a car the same distance as one acre of solar panels powering an EV.
The same episode also notes that devoting one acre of land to corn ethanol yields much less carbon benefit than devoting that same acre to forest.
Another analysis claims that producing sustainable jet fuel from corn or soybeans will use 16 or 70 times as much land (respectively) as would be needed to produce the same amount of fuel from solar power.
A 2011 publication from the RAND Corporation finds that “on an equivalent energy basis, most biomass in most regions is more expensive than coal”, and coal is already being outcompeted in energy markets, even without taking into account its negative externalities (many of which are shared by biomass).
As summarized by an IEA report, IPCC net zero scenarios for 2050 “use a median of 200 EJ of bioenergy in 2050”, with some scenarios exceeding 300 EJ. Another source projects total world energy usage in 2050 at just under 800 EJ; 200 EJ of bioenergy would be 25% of that total. These figures are not necessarily comparable, but it’s clear that bioenergy is playing a large role in these scenarios.
This is a rough estimate, potentially failing to take into account conversion losses and other important factors.
For one thing, biomass varies from place to place – by type, moisture content, etc. – and so it’s hard to deploy standardized, mass-produced processing facilities. One source notes, “In forest biomass supply chains, it is difficult to achieve the same economies of scale as are possible with fossil fuel extraction”.
Very good piece, very good. I like the art work, too. Two minor points You used the EJ unit as if readers are all familiar with it, but I had to look it up, learned that there are about 1050 J/btu, then realized I have no idea of the units by which to measure world energy conversion and therefore no idea of how 50 EJ from biomass compares to some other energy conversion process, e.g. PV solar electricity in a certain future year. Could you give readers such a comparison?
The question of burning waste forest products from a managed young forest in which every tree felled has a seedling planted by the stump has bugged me for months. Is it C neutral or not? You point out that burning wood waste empties a sink. When I asked this of an expert who was kind enough to reply to the email equivalent of a cold call, he wrote back that it is C-neutral "because the log would eventually have rotted." [and thus given up its CO2 to the air over some years]. From his reply I infer that the only way wood dropped to the ground can be a true long-term sink is when it's permanently underground, becoming soil. Burning forest product waste for fuel can only be seen as C neutral when the forest is managed by the best available methods, which may be more theoretical than real.
Please remind me, Steve, how I can share your excellent blogs with friends who are not subscribers
Thank you, Steve, for follow-up on EJ.
It's unlikely any forests are "managed" optimally. Whether burning forest product waste can truly be carbon-neutral is more a thought experiment than a real-world consideration, especially when the emissions involved in creating the waste (i.e. felling the trees, de-branching them and transporting the waste to the burn site) are counted. I have shared your blog on twitter and a slack group.