You’ll notice something new today: instead of stock photography, we have beautiful illustrations by Nicole Kelner. I’ll be working with a few different illustrators in the coming weeks. Let me know what you think!
You’re probably tired of hearing about the astonishing cost declines for clean solar, wind, and battery power. And that’s not all; for more and more products, the “green premium” is vanishing, as non-greenhouse-gas-emitting solutions become cheaper, or otherwise superior, to their polluting counterparts. This is probably the single largest reason for climate optimism.
However, not all products come down rapidly in price. For instance, the cost of nuclear power has actually increased in recent decades, at least in the US. What gives?
A recent paper, Accelerating Low-Carbon Innovation, helps explain which technologies are likely to experience rapid cost decline. This can help us understand which technologies are good bets for future success. It also shows that government actions, such as subsidies, won’t always be able to make a technology become successful.
The authors of this paper were recently interviewed on the (always recommended) Volts podcast, during which they shared a lot of additional context. In today’s post, I’m drawing on both the paper and the interview.
Some Technologies Progress Faster Than Others
The basic idea is simple: over time, as we manufacture more solar panels or nuclear power plants or whatever, we naturally find opportunities to reduce cost. A variety of factors influence the rate of progress:
Standardization. Solar cells are pretty much the same whether they’re on a rooftop in the US or a utility-scale installation in India, so an innovation developed anywhere in the vast solar cell manufacturing industry can quickly be applied everywhere. However, the design of a nuclear power plant varies according to size, site, and local regulations, so ideas discovered during one project may only be applicable to that project. To the extent that a product needs to be adapted to customer preferences, regulation, or the physical environment, that reduces potential for improvements to propagate from one market niche to another.
Product complexity. Simpler products, like solar panels, are easier to optimize. It’s also relatively easy to optimize a product that has many components if they are only loosely coupled, such as a laptop or smartphone, where updating one component doesn’t affect others – you can update the camera without having to redesign the battery. In a nuclear plant, by contrast, modifying one component will likely require adjusting the entire design. I imagine there are a couple of reasons that product complexity affects cost reductions:
Solar panels have only a few components, so if you find a way to improve one component, you’ve made a substantial contribution. A nuclear plant has many components, so an improvement to any one component has less impact.
The tight coupling of components in a nuclear reactor makes it hard to improve anything – you need to consider the impact on the entire system. From the interview: “complex products have a lot of nonlinear interactions between subcomponents”, making it harder to get anything done.
Manufacturing complexity. “A certain level of complexity in the manufacturing process is needed to see high learning rates.” I imagine the underlying principle here is that if the manufacturing process is simple, then you quickly run out of things to optimize. Lumber doesn’t get cheaper, because it’s already as cheap as it’s going to get. But in the case of solar cells, while the end product is simple, the manufacturing process is not, and there is room for optimization – for instance, by finding ways to slice the expensive silicon ingots more thinly.
Barriers to entry. If a product is very complex, and/or comes in large, expensive units (a single conventional nuclear plant costs billions of dollars), that creates a barrier to competition: it’s very hard for a new competitor to find the money to enter the market. This reduces competitive pressure to find cost savings.
Project timeline. Large, complex projects take a long time to design, implement, and deploy, so learning happens at a slower pace. In the case of a nuclear power plant, the time from the initial feasibility study through design, permitting, site preparation, construction, and startup can be ten years. That means it takes ten years to test a new idea. The time to manufacture a solar panel, or even to build an entirely new factory, is much shorter, so improvements can happen on a faster timeline.
Market growth. As costs for a given product come down, it becomes harder and harder to find the next improvement. If the market is growing, then the increased size of the industry drives both economies of scale and higher R&D budgets, allowing cost reductions to continue. This is why booming industries such as solar panels or computer chips continue to wring out efficiency gains, decade after decade after decade.
The authors also note that we don’t generally see learning curves for products which are based on digging stuff out of the ground, i.e. raw materials.
The Power of Standards
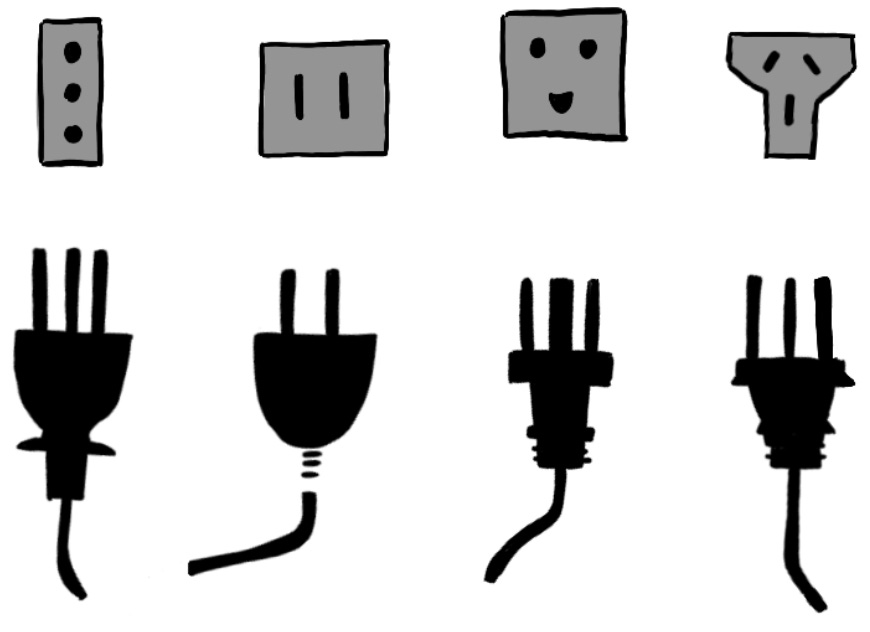
By identifying the factors that cause technologies to come down in price, the paper shines a light on ways to accelerate the process. The first approach I’ll discuss is standardization.
Governmental policy at the local, national, and international level can all influence the level of standardization. This doesn’t necessarily mean enforcing a specific standard, though it can – by the end of 2024, all mobile phones sold in the EU will have to use the same USB-C charging plug. That’s being done for the convenience of consumers, but such standards also reduce the need for manufacturers to create a different product for each market.
In many cases, rather than requiring standardization, governments actually forbid it. Variations in building and transportation codes force products to be customized to each market. By harmonizing standards across regions and countries, we can promote standardization without relying on slow-moving government agencies to actually mandate a specific standard.
Streamlining regulatory processes also helps, by reducing project timelines and thus speeding up the innovation cycle. And if a market lacks for competition, governments may be able to reduce barriers to entry by removing legal hurdles and subsidizing early investment.
If regulations change over time, that can be even more disruptive than differences between countries. One example is nuclear safety rules in the US, which have long been a moving target; this impedes cost optimization from one project to the next.
Of course, there are tradeoffs to all of this. If we enforce a standard too early, that can block innovation. For instance, EV manufacturers have been finding better and better ways to integrate battery packs into the structure of a car. If the government had imposed an early standard on battery pack design, those improvements wouldn’t have been possible. This is why it may be safer to focus on eliminating code variations, without taking the further step of mandating a specific standard. But sometimes that further step is called for.
In the early stage of large, complex technologies, where individual countries can’t afford to run their own demonstration projects, international cooperation becomes important. In these cases, we need shared projects where the learning can be widely disseminated. Of course this is in tension with the desire for proprietary advantage, which has been an issue, for example, in CCS (Carbon Capture and Storage) technology, and will also likely be an issue for advanced nuclear designs. For instance, France and South Korea have historically managed to achieve modest cost designs on nuclear power, through the use of standardized designs, but this benefit has not propagated to other countries. Quoting from the paper:
…[The] Paris Agreement’s reliance on national green industrial policies is suited for promoting innovation only for some technologies. For highly complex and/or customized technologies, such as nuclear power, biomass power, and CCS, national policies need to be augmented with top-down measures to coordinate research, development, demonstration, deployment, and regulations across countries to facilitate learning-by-doing and inter-project and inter-context spillovers at a regional or global scale.
Subsidies Only Work When There’s a Learning Curve
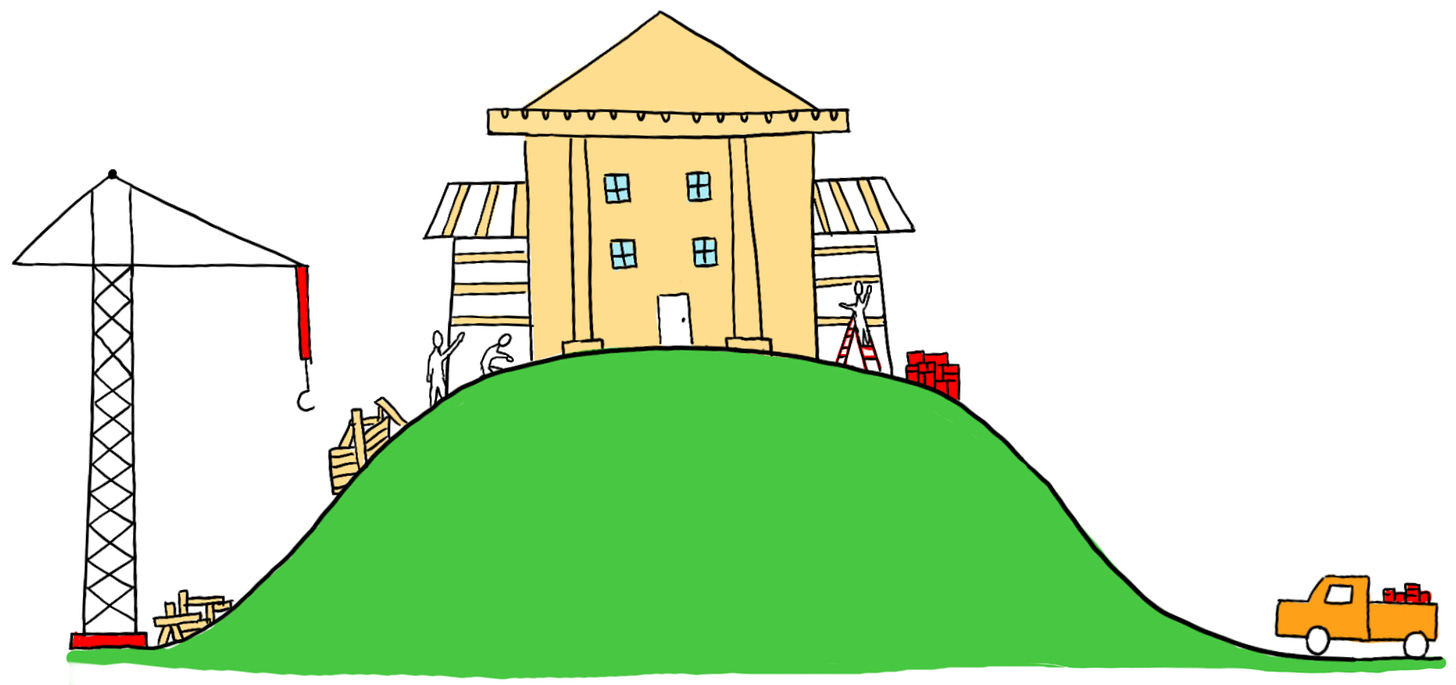
If a technology is amenable to a steep learning curve – if it is standardized, simple, has short project timelines, and so forth – then costs will come down almost automatically as a side effect of increased usage. This is where subsidies, purchasing requirements, and other incentives can have an impact. Famously, early subsidies for solar panels – in Germany, for instance – carried the industry past its early period of high costs and allowed solar power to become competitive without subsidies.
Conversely, technologies that are not amenable to learning curves may not be good candidates for subsidy. The cost of nuclear power has not been decreasing with each new plant, so it’s unlikely that subsidizing more plants would bring costs down.
Which Specific Technologies Will Do Well
The paper provides a nice chart to organize different technologies. “Type 1” technologies in the lower-left corner show the steepest cost declines; “type 3” technologies along the top and right sides benefit the least.
Note that BECCS and biomass are in the “Customized” column in part because the feedstock varies from place to place. Biomass varies from place to place – by type, moisture content, etc. – and so it’s hard to design a standardized, mass-produced processing facility.
Implications for Climate Change Mitigation
Ongoing price declines have the potential to accelerate the transition to clean energy sources. Current models from the IPCC, IEA, and other organizations often either fail to take learning curves into account, or assume that all technologies will improve at the same rate. In the real world, technologies with steep learning curves – such as batteries and solar panels – will play a larger role than the models show, and may help the transition move faster than expected. In particular, once these simple, standardized “type 1” technologies become economically viable without subsidies, adoption accelerates rapidly, as a virtuous cycle emerges: increased sales → lower cost → increased sales. (In hindsight, it’s remarkable that subsidies for solar power were sustained across the very long time period required for the technology to finally become cost competitive.)
By the same token, we should be wary when models envision widespread use of complex / customized Type 3 technologies, such as BECCS or nuclear power. If those technologies aren’t economical today, they’re going to have a hard time getting there in the future.
Across all technologies, we can accelerate progress by harmonizing regulations and removing other barriers to standardization. For Type 3 technologies, international cooperation on technology development, demonstration projects, and innovation sharing are critical. And we should not expect subsidies to drive cost reduction for nuclear power, BECCS, or other type 3 technologies.